A scanning electron microscopic lense image reveals branched fractures in a single crystal of 2D hexagonal boron nitride (h-BN). Experiments and computational modelling by Rice University and Nanyang Technological University revealed h-BN lattice asymmetry permits fractures to follow branching courses, which efficiently strengthens the 2D product by making it harder for fractures to propagate. Credit: Image thanks to J. Lou/Rice University
2D product withstands breaking and description by century-old theory of fracture mechanics.
It’s authorities: Hexagonal boron nitride (h-BN) is the iron guy of 2D products, so resistant to breaking that it defies a century-old theoretical description engineers still utilize to determine durability.
“What we observed in this material is remarkable,” stated Rice University’s Jun Lou, co-corresponding author of a Nature paper released today. “Nobody expected to see this in 2D materials. That’s why it’s so exciting.”
Lou describes the significance of the discovery by comparing the fracture durability of h-BN with that of its better-known cousin graphene. Structurally, graphene and h-BN are almost similar. In each, atoms are organized in a flat lattice of adjoining hexagons. In graphene, all the atoms are carbon, and in h-BN each hexagon includes 3 nitrogen and 3 boron atoms.
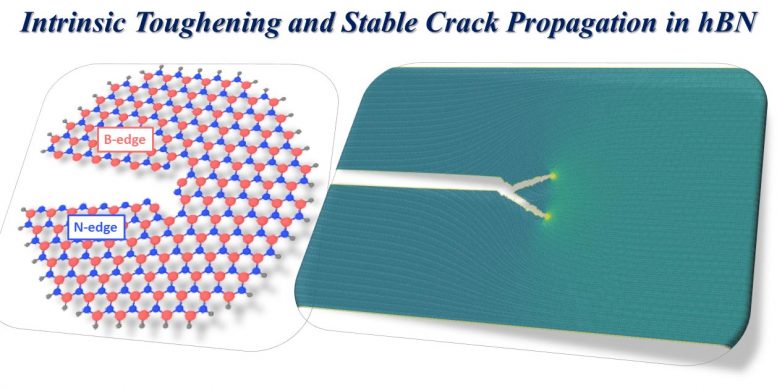
Computational simulations at Nanyang Technological University in Singapore assisted discuss the unanticipated fracture durability of 2D hexagonal boron nitride. The product’s intrinsic durability develops from minor asymmetries in its atomic structure (left), which produce an irreversible propensity for moving fractures to follow branched courses (right). Credit: Image thanks to H. Gao/NTU
The carbon-carbon bonds in graphene are nature’s greatest, which must make graphene the most difficult things around. But there’s a catch. If even a couple of atoms run out location, graphene’s efficiency can go from amazing to average. And in the real life, no product is defect-free, Lou stated, which is why fracture durability — or resistance to split development — is so crucial in engineering: It explains precisely just how much penalty a real-world product can stand up to prior to stopping working.
“We measured the fracture toughness of graphene seven years ago, and it’s actually not very resistant to fracture,” Lou stated. “If you have a crack in the lattice, a small load will just break that material.”
In a word, graphene is breakable. British engineer A.A. Griffith released an influential theoretical research study of fracture mechanics in 1921 that explained the failure of breakable products. Griffith’s work explained the relationship in between the size of a fracture in a product and the quantity of force needed to make the fracture grow.
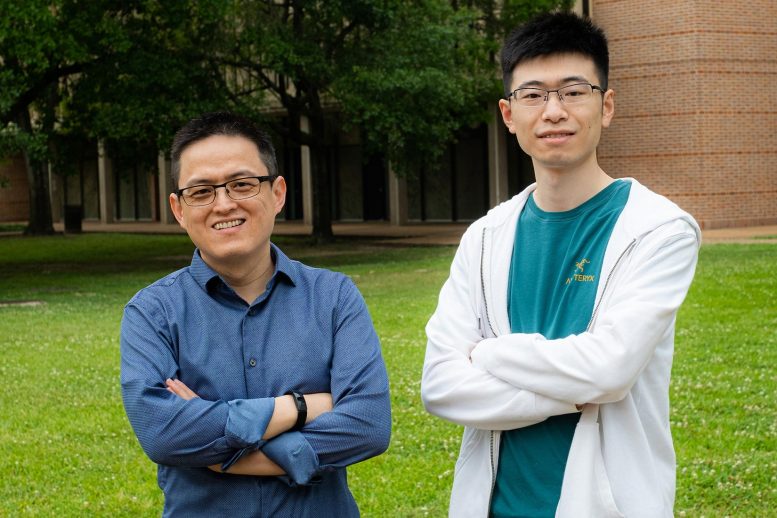
Experiments by Rice University products researchers Jun Lou (left) and Boyu Zhang revealed 2D hexagonal boron nitride was remarkably resistant to breaking. Credit: Photo by Jeff Fitlow/Rice University
Lou’s 2014 research study revealed graphene’s fracture durability might be discussed by Griffith’s tried and true requirement. Given h-BN’s structural resemblances to graphene, it likewise was anticipated to be breakable.
That isn’t the case. Hexagonal boron nitride’s fracture resistance has to do with 10 times greater than graphene’s, and h-BN’s habits in fracture tests was so unanticipated that it defied description with Griffith’s formula. Showing specifically how it acted and why took more than 1,000 hours of experiments in Lou’s laboratory at Rice and similarly painstaking theoretical work headed by co-corresponding author Huajian Gao at Nanyang Technological University (NTU) in Singapore.
“What makes this work so exciting is that it unveils an intrinsic toughening mechanism in a supposedly perfectly brittle material,” Gao stated. “Apparently, even Griffith couldn’t foresee such drastically different fracture behaviors in two brittle materials with similar atomic structures.”
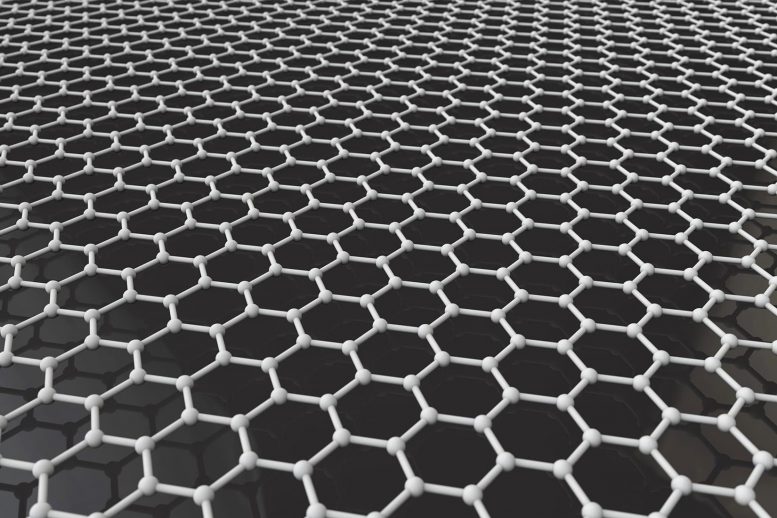
In both graphene and hexagonal boron nitride (h-BN) atoms, atoms are organized in a flat lattice of adjoining hexagons. In graphene, all the atoms are carbon. In h-BN each hexagon includes 3 nitrogen and 3 boron atoms. Credit: Image thanks to Rice University
Lou, Gao and associates traced the extremely various product habits to minor asymmetries that arise from h-BN including 2 aspects rather of one.
“Boron and nitrogen are not the same, so even though you have this hexagon, it is not exactly like the carbon hexagon (in graphene) because of this asymmetric arrangement,” Lou stated.
He stated the information of the theoretical description are intricate, however the result is fractures in h-BN tend to branch and turn. In graphene, the pointer of the fracture takes a trip directly through the product, opening bonds like a zipper. But the lattice asymmetry in h-BN produces a “bifurcation” where branches can form.
“If the crack is branched, that means it is turning,” Lou stated. “If you have this turning crack, it basically costs additional energy to drive the crack further. So you’ve effectively toughened your material by making it much harder for the crack to propagate.”
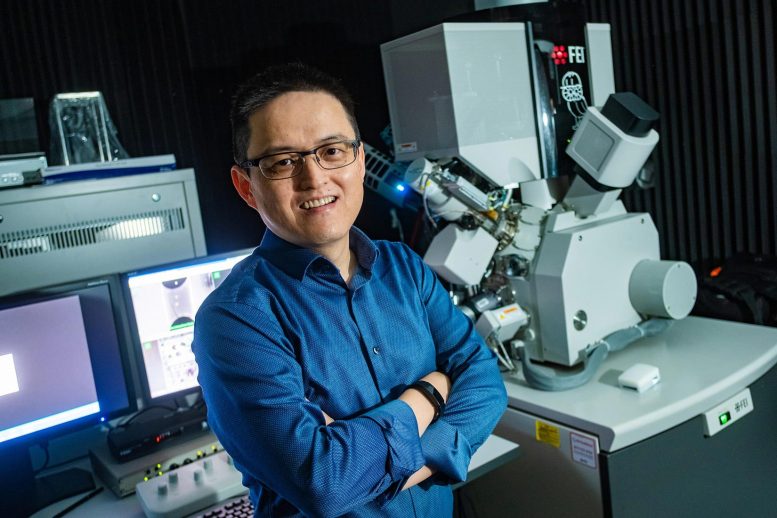
Jun Lou. Credit: Photo by Jeff Fitlow/Rice University
Gao stated, “The intrinsic lattice asymmetry endows h-BN with a permanent tendency for a moving crack to branch off its path, like a skier who has lost her or his ability to maintain a balanced posture to move straight forward.”
Hexagonal boron nitride is currently an exceptionally crucial product for 2D electronic devices and other applications since of its heat resistance, chemical stability and dielectric homes, which enable it to work as both a supporting base and an insulating layer in between electronic parts. Lou stated h-BN’s unexpected durability might likewise make it the perfect choice for including tear resistance to versatile electronic devices made from 2D products, which tend to be breakable.
“The niche area for 2D material-based electronics is the flexible device,” Lou stated.
In addition to applications like electronic fabrics, 2D electronic devices are thin enough for more unique applications like electronic tattoos and implants that might be connected straight to the brain, he stated.
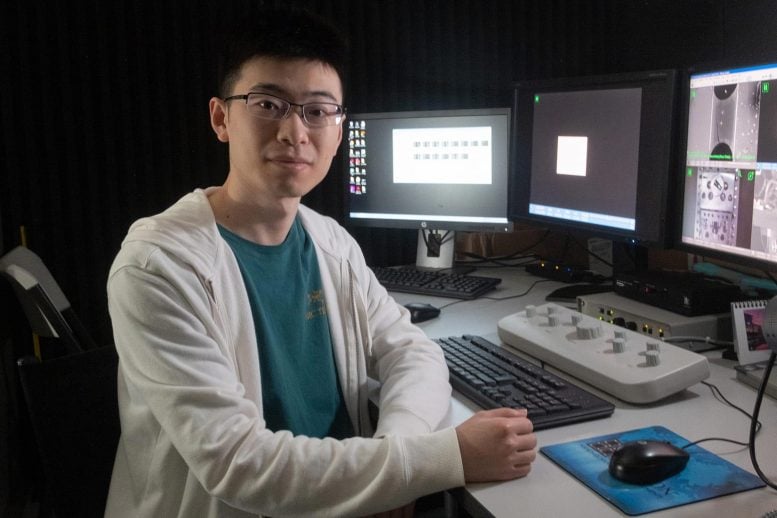
Boyu Zhang. Credit: Photo by Jeff Fitlow/Rice University
“For this type of configuration, you need to ensure the material itself is mechanically robust when you bend it around,” Lou stated. “That h-BN is so fracture-resistant is great news for the 2D electronic community, because it can use this material as a very effective protective layer.”
Gao stated the findings might likewise indicate a brand-new path to produce hard mechanical metamaterials through crafted structural asymmetry.
“Under extreme loading, fracture may be inevitable, but its catastrophic effects can be mitigated through structural design,” Gao stated.
Lou is a teacher and partner department chair in products science and nanoengineering and a teacher of chemistry at Rice. Gao is a recognized university teacher in the schools of both engineering and science at NTU.
Rice-associated co-authors are Yingchao Yang, now an assistant teacher at the University of Maine, Chao Wang, now at the Harbin Institute of Technology in China, and Boyu Zhang. Other co-authors consist of Bo Ni of Brown University; Xiaoyan Li of Tsinghua University in China; Guangyuan Lu, Qinghua Zhang, Lin Gu and Xiaoming Xie of the Chinese Academy of Sciences; and Zhigong Song of the Agency for Science, Technology and Research in Singapore and previously of Tsinghua and Brown.
Reference: “Intrinsic toughening and stable crack propagation in hexagonal boron nitride” by Yingchao Yang, Zhigong Song, Guangyuan Lu, Qinghua Zhang, Boyu Zhang, Bo Ni, Chao Wang, Xiaoyan Li, Lin Gu, Xiaoming Xie, Huajian Gao and Jun Lou, 2 June 2021, Nature.
DOI: 10.1038/s41586-021-03488-1
The research study was supported by the Department of Energy (DE-SC0018193), and simulations were carried out on resources offered by the National Science Foundation’s Extreme Science and Engineering Discovery Environment (MSS090046) at Brown University’s Center for Computation and Visualization.